harold fellermann
Complex Systems Modelling
Simbiotics: a multi-scale integrative platform for 3D modeling of bacterial populations
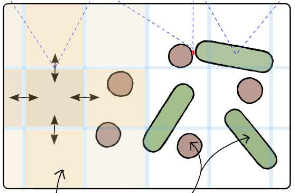
Simbiotics is a spatially explicit multi-scale modeling platform for the design, simulation and analysis of bacterial populations. Systems ranging from planktonic cells and colonies, to biofilm formation and development may be modeled. Representation of biological systems in Simbiotics is flexible, user-defined processes may be in a variety of forms depending on desired model abstraction. Simbiotics provides a library of modules such as cell geometries, physical force dynamics, genetic circuits, metabolic pathways, chemical diffusion and cell interactions. Model defined processes are integrated and scheduled for parallel multi-thead and multi-CPU execution. A virtual lab provides the modeler with analysis modules and some simulated lab equipment, enabling automation of sample interaction and data collection. An extendable and modular framework allows for the platform to be updated as novel models of bacteria are developed, coupled with an intuitive user interface to allow for model definitions with minimal programming experience. Simbiotics can integrate existing standards such as SBML, and process microscopy images to initialise the 3D spatial configuration of bacteria consortia. Two case studies, used to illustrate the platform flexibility, focus on the physical properties of the biosystems modeled. These pilot case studies demonstrate Simbiotics versatility in modeling and analysis of natural systems and as a CAD tool for synthetic biology.
J. Naylor, H. Fellermann, Y. Ding, W. K. Mohammed, N. S. Jakubovics, J. Mukherjee, C. A. Biggs, P. C. Wright, & N. Krasnogor, ACS Synthetic Biology, 2017
Formalising Modularisation and Data Hiding for Synthetic Biology
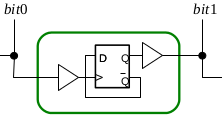
Biological systems employ compartmentalisation and other co-localisation strategies in order to orchestrate a multitude of biochemical processes by simultaneously enabling "data hiding" and modularisation. This paper presents recent research that embraces compartmentalisation and co-location as an organisational programmatic principle in synthetic biological and biomimetic systems. In these systems, artificial vesicles and synthetic minimal cells are envisioned as nanoscale reactors for programmable biochemical synthesis and as chassis for molecular information processing. We present P systems, brane calculi, and the recently developed chemtainer calculus as formal frameworks providing data hiding and modularisation and thus enabling the representation of highly complicated hierarchically organised compartmentalised reaction systems. We demonstrate how compartmentalisation can greatly reduce the complexity required to implement computational functionality, and how addressable compartments permits the scaling-up of programmable chemical synthesis.
H. Fellermann, M. Hadorn, R. Füchslin & N. Krasnogor, ACM J. Emerg. Tech. Comp. Sys 11(3):24, 2014
H. Fellermann & N. Krasnogor, Lect. Notes Comp. Sci. 8493:173-182, 2014
Modelling and stochastic simulation of synthetic biological Boolean gates
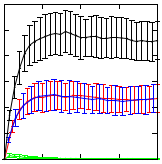
Synthetic Biology aspires to design and compose biological systems that implement specified behaviour in engineered biological system. When designing such systems, hypothesis testing via computational modelling and simulation is vital in order to reduce the need of costly wet lab experiments. As a case study, we here discuss the use of computational modelling and stochastic simulation of engineered genetic circuits that implement Boolean AND and OR gates that have been reported in the literature. We present performance analysis results for nine different state-of-the-art stochastic simulation algorithms and analyse the dynamic behaviour of the proposed gates. Stochastic simulations verify the desired functioning of the proposed gate designs.
D. Sanassy, H. Fellermann, N. Krasnogor, S. Konur, L. M. Mierla, M. Gheorghe, C. Ladroue & S. Kalvala, Proceedings of the 2014 IEEE International Conference on High Performance Computing and Communications pp. 404-408
Programming Chemistry in DNA Adressable Bioreactors
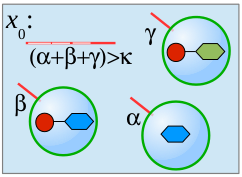
We present a formal calculus, termed chemtainer calculus, able to capture the complexity of compartmentalized reaction systems such as populations of possibly nested vesicular compartments. Compartments contain molecular cargo as well as surface markers in the form of DNA single strands. These markers serve as compartment addresses and allow for their targeted transport and fusion, thereby enabling reactions of previously separated chemicals. The overall system organization allows for the setup of programmable chemistry in microfluidic or other automated environments. We introduce a simple sequential programming language whose instructions are motivated by state-of-the-art microfluidic technology. Our approach integrates electronic control, chemical computing, and material production in a unified formal framework that is able to mimick the integrated computational and constructive capabilities of the subcellular matrix. We provide a non-deterministic semantics of our programming language that enables us to analytically derive the computational and constructive power of our machinary. This semantics is used to derive the sets of all constructable chemicals and supermolecular structures that emerge from different underlying instruction sets. Since our proofs are constructive, they can be utilized to automatically infer control programs for the construction of target structures from a limited set of resource molecules. Finally, we present an example of our framework from the area of oligosaccharide synthesis.
H. Fellermann & L. Cardelli, R. Soc. Interface 11(99), 2014
Coarse graining and scaling in dissipative particle dynamics
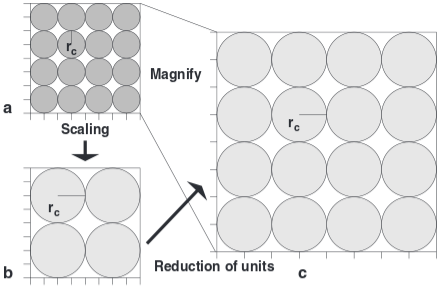
Dissipative particle dynamics (DPD) is now a well-established method for simulating soft matter systems. However, its applicability was recently questioned because some investigations showed an upper coarse-graining limit that would prevent the applicability of the method to the whole mesoscopic range. This article aims to re-establish DPD as a truly mesoscopic method by analyzing the problems reported by other authors and by presenting a scaling scheme that allows one to apply DPD simulations directly to any desired length scale.
R. Füchslin, H. Fellermann, A. Eriksson & H.-J. Ziock, J. Chem. Phys. 130(21):214102, 2009